Listen to the Music not the Room originally written by Dr. Peter D’Antonio of RPG Difuser Systems
Room reflections cause acoustic distortion
The audio product created in a studio needs to sound similar in a wide range of listening environments. Thus the audio product must be transferable to these different environments.
The engineer must be aware of the acoustic signature of the room in which the audio product is created so that the room’s acoustic signature is not embedded into the audio product.
Since we have no control over the acoustic design of the environments into which the audio product will be transferred, every effort should be made to provide good acoustics in the creation environment so that the necessary image and signal balances can be executed correctly so that countless hours creating the product are not wasted.
The recording industry is conscious of electronic distortion, but acoustic distortion is often overlooked in the pursuit of more electronic equipment.
Experience the difference – LISTEN TO THE MUSIC NOT THE ROOM
When the sound from a loudspeaker encounters the boundaries of a room, a very complex series of reflections occur. It is very difficult to isolate the direct sound alone, because these reflections interact with it, and among themselves, to produce a wide range of effects, which we call acoustic distortion.
If proper acoustic design is not utilized, a room will introduce sonic distortion. This prevents the engineer from hearing all of the detail information the loudspeakers and electronics are capable of delivering.
The Acoustic Distortion introduced by the room can be so influential that it dominates the overall sonic impression. The causes of acoustic distortion are:
-
- Modal Coupling – the acoustical coupling between the speakers and listener with the room’s modal pressure variations or room modes.
-
- Speaker-Boundary Interference – the coherent interaction between the direct sound and the omni-directional early reflections from the room’s adjacent boundaries.
-
- Comb Filtering – the coherent constructive and destructive interference between the direct sound and early reflections.
-
- Sound Diffusion – the spatial and temporal reflection pattern due to mid and late arriving reflections.
When one stops and realises that the speaker/room interface is your acoustic microscope, it seems prudent to strive for the ultimate sonic resolution.
Pound for pound the acoustic treatment in a room will make more of an audible difference than any piece of electronic hardware, speaker, or cable.
Acoustic Distortion affects the Perception of Sound
Acoustic distortion affects three psychoacoustic perceptions, namely:
Frequency Response or Timbre – refers to the perception of the harmonic content of the music and its spectrum.
Imaging – refers to the size, and apparent position, of sonic images in the stereo sound-stage.
Spatial Impression – is the sense of spatiality, envelopment or sense of immersion in the sonic experience. All three forms of distortion occur across the entire frequency spectrum, but room modes and the speaker/boundary interactions predominate at low frequencies.
Electronic equivalent of room colouration
Imagine secretly inserting a parametric equaliser and digital delay unit into the signal path prior to a critical mix with the circuit set to boost:
71Hz, 142Hz and 213Hz by 10dB
and introduce 4ms, 7ms and 10ms of delay.
The engineer would mix the sound and subconsciously attempt to adjust for these effects. Imagine the engineer’s surprise when they were informed of the trick and they played back the mix with the equaliser and delay switched off!
This is not a practical joke because the room is playing tricks on the engineer – all the time. The engineer will always be mixing wearing ‘room coloured’ ear muffs.
Room Modes
Sound waves reinforce and cancel each other as they reflect back and forth between hard walls. This constructive/destructive interference results in resonances at frequencies determined by the distances between the walls.
For example, a 2.44m floor to ceiling dimension results in a fundamental room mode of 71Hz. This modal response is characterised by high and low pressure zones throughout the room. The loudspeaker placement will accentuate or diminish modal frequencies depending on placement. Similarly, an engineer will hear different modal responses depending on where they are seated.
Figure 1 illustrates how the sound energy is distributed along a room dimension. The room dimension is shown as a fraction ranging from 0 to 1. Then 0.5 would be in the centre of the room and 1 would be against a wall. Examining Figure 1 reveals that the fundamental has no energy in the centre of the room.
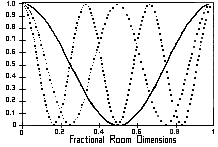
Physically this means that if you were sitting in the middle of the room you would not hear this frequency.
The first harmonic, however, is at a maximum. It can be inferred from this graph that, in the centre of the room, all even harmonics are absent and all odd harmonics are at a maximum.
When listening to music in a room, the music will be modified by the room’s modal response and this acoustic distortion will depend on where the speakers and the engineer are located.
As an example, Figure 2 presents the modal frequency response of a room with a 4.57m dimension. The microphone is located against a wall perpendicular to this dimension so that all modes along this dimension will be present.
The first three modes are identified as (1,0,0), fundamental, (2,0,0), first harmonic and (3,0,0), second harmonic. At low frequencies the room modes are widely separated and present a potential problem.
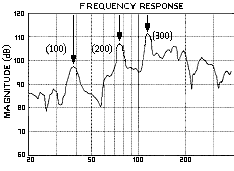
As the frequency increases, room modes are still present, but their number and density increase and are not perceived as a problem. This form of modal acoustic distortion is best addressed with low frequency absorption.
One can easily simulate the effect of room modes by maximizing a parametric equalizer at only specific frequencies associated with the room’s dimensions. If the room did not introduce any modification to what is being heard, Figure 2 would be a flat line.
Speaker-Boundary Interference Response
This distortion occurs across the entire frequency spectrum, but is more significant at low frequencies. We refer to it as the Speaker Boundary Interference Response or SBIR.
In Figure 3 a loudspeaker is located 0.9m from each room surface with coordinates (3,3,3). The four virtual images responsible for first order reflections are also shown, one on the opposite side of the main room boundaries. A virtual image is located an equivalent distance on the opposite side of a room boundary.
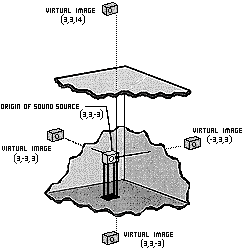
The distance from a virtual source to the listener is equal to the reflected path from source to listener. In addition to the four virtual images shown, there are seven more.
Three virtual images and one real image in the speaker plane and four images of these above the ceiling and floor planes.
Imagine that the walls are removed and 11 additional physical speakers are located at the virtual image positions. The resultant sound at a listening position would be equivalent to the sound heard.
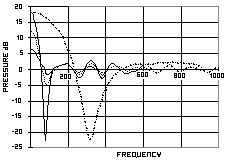
The effect of the coherent interference between the direct sound and these virtual images is illustrated in Figure 4. The SBIR is averaged over all listening positions with the speaker located 4ft from one, two and three walls surrounding the loudspeaker.
It can be seen that as each wall is added, the low frequency response increases by 6dB and the notch, at roughly 100Hz, gets deeper. At low frequencies there is typically very little absorption efficiency on the boundary surfaces and the notches can be between 6 and 2dB.
As in the case of room modes, The SBIR response in Figure 4 would be a flat line at 0dB. The conclusion is obvious:
Never place a speaker’s woofer equidistant from the floor and two surrounding walls. The low frequency rise illustrates why one can add ore bass by moving the speakers into the corners of a room.
Comb Filtering
Another form of acoustic distortion introduced by room reflections is comb filtering. It is due to interference between the direct sound and a reflected sound. In Professional Control Rooms, we are primarily concerned with the interaction between the direct sound and the first-order (i.e. single-bounce) reflections.
Reflections cause a time delays, because the reflected path length between the Engineer and source is longer than the direct sound path. Thus when the direct sound is combined with the reflected sound, we experience notching and peaks referred to as comb filtering.
The location of the first notch is given by the speed of sound divided by twice the total path length difference. The spacing between subsequent notches is twice this frequency. For example, a 1ms delay corresponds to a path length difference of 0.34m.
The first notch occurs at 500Hz, with subsequent notches 1000Hz apart. The audible effect of comb filtering is easy to experience using a delay line and produces various effects such as chorusing or flanging, depending on the length of the delay.
Shorter delays have wider bandwidth notches and thus remove more power than longer delays. This is why microsecond and millisecond delays are so audible. The effect of a reflection is illustrated in Figure 5 and Figure 6.
In Figure 5 we illustrate the time and frequency responses from the right speaker only. The upper curve shows the arrival time and the lower curve shows the free-field response of the loudspeaker.
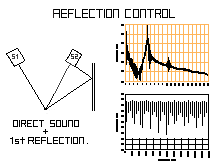
In Figure 6 we show the effect of adding a side wall reflection to the sound of the right speaker. Now the upper curve shows the arrival time of both the direct sound and the reflected sound.
The lower curve shows the severe comb filtering that a single reflection introduces.
If a speaker had a free-field response like this lower curve, it would never be accepted. Yet, many rooms are designed without reflection control.
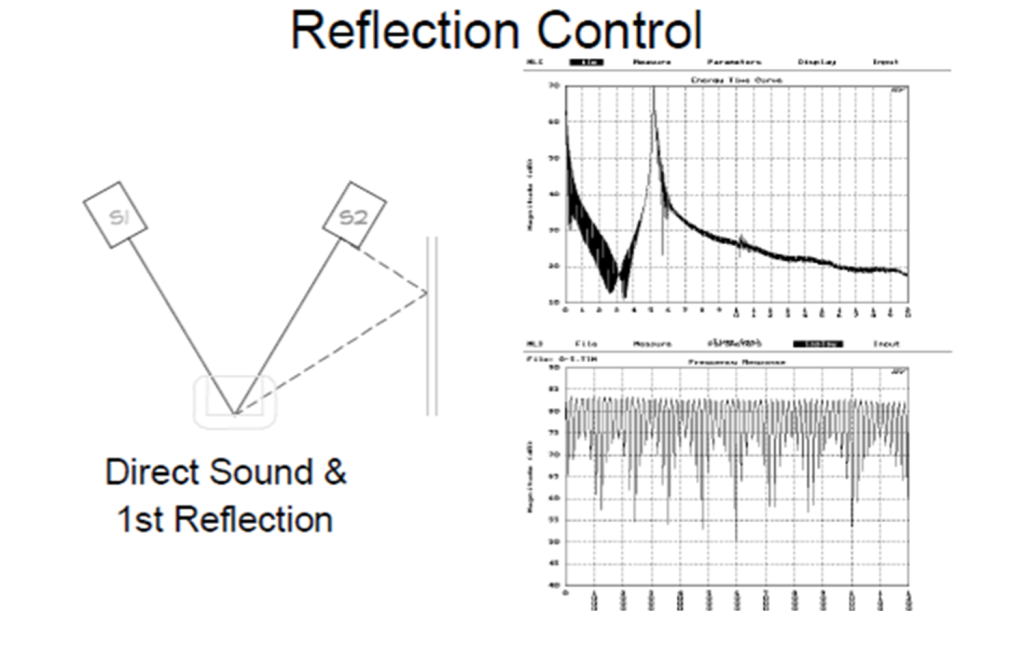
The message is to absorb these interfering reflections so we can listen to the music, and not the room.
Imaging
Imaging refers to our ability to perceive and accurately locate the instruments, voices and effects which comprise the sound-stage. The factors affecting localization and the acoustic sound-stage are reflections from the room’s boundary surfaces which cause comb filtering.
These reflections cause frequency response notches and peaks which fool the ear/brain (auditory system) and degrade our ability to experience the sonic images as they were intended to be perceived. Imaging is optimized by an Imaging Acoustic Treatment which absorbs the room’s first order reflections over a wide range of frequencies.
Spatial Impression
The spatial impression refers to the intangible sensation of openness, envelopment or spaciousness and the feeling that we are part of or immersed in the audio/video event we are experiencing. Good Control Rooms allow us to faithfully recreate an experience.
The sense of envelopment is related to the amount of sound reaching the mix position from the sides of the room. The use of sound diffusing surfaces on the rear wall help to naturally create an impression of ‘passive surround sound’.
Thus Diffusive Acoustic Treatment is used to create envelopment, widen the sweet spot, add warmth and naturalness to the sound, and uniformly distribute the sound throughout the room.
Frequency Response (Bass Response)
The perceived bass response in a room is controlled by:
-
- free-field speaker response.
-
- room dimensions.
-
- acoustic coupling of the engineer/speakers to the rooms modal pressure variations.
-
- speaker-boundary interference between the direct sound and adjacent reflections.
-
- internal contents of the room.
-
- acoustic nature of the boundary surfaces and surface treatment.
-
- hearing response and training of the engineer. Any absorption or amplification over a frequency. range that is introduced by the room will colour the sound that we hear. The room will thus introduce its own signature rather than having a flat frequency response.
Since most of today’s speakers are of high quality and we may not have any control over the room’s dimensions, we can focus our attention on proper speaker placement and acoustic treatment.
Proper speaker placement can minimize agitation of the room’s resonant frequencies and the interference between the speaker and reflected sounds from the nearby corner. This can result in severe cancellations if the speaker is located an equal distance from all boundaries.
After locating the speakers (and engineer) properly, Bass Absorption should be used to reduce the room resonances and minimize the speaker/boundary interference.
Damp the Modes
The magnitude of the room mode effects can be reduced by increasing the damping factor of all the modes by suitable use of low frequency absorbers. The most common are acoustical foam or other porous absorbers which absorb sound by converting sound energy into heat.. The efficiency of a porous absorber is highest when the sound is traveling at its highest velocity. This point is reached at ¼ of the wavelength and thus varies with frequency. At low frequencies like 100Hz, this distance is about 750mm from the wall. Unfortunately at the wall surface where the porous absorber is usually placed, the particle velocity is zero.
This is where the sound waves change direction on reflection and hence the velocity is zero. Since porous absorbers rely on particle velocity, they have minimal efficiency at low frequencies.
The points to be taken away are that:
-
- PLACING POROUS ABSORBERS ON A WALL IS POINTLESS
2. ACOUSTIC TREATMENTS MINIMISE ACOUSTIC DISTORTION AND IMPROVE THE PERCEPTION OF SOUND.
Summary
-
- If you can’t take the room out of the mix, you can take the mix out of the room.
-
- If you are building a new room, use good dimensional ratios to provide uniform modal frequency distribution.
-
- Design for a symmetrical listening environment for good imaging. Place the speakers symmetrically and on axis for the best response.
-
- Locate the speaker and engineer’s position to optimise the acoustic coupling with the room’s pressure variations and speaker-boundary interference. i.e. optimise the bass response.
-
- Minimise first order reflections from the walls, ceiling and floor between the speakers and the listening position using appropriate absorption or diffusion.
-
- BEWARE of console reflections and minimise them.
-
- Diffuse rear wall reflections over at least 60% of the surface.
-
- Provide low frequency absorption on the rear wall to minimise low frequency cancellation effects.
-
- Damp low frequency modes by applying appropriate low frequency absorbers at maximum room pressure locations.
CAUTION
Absorption coefficients of many absorbers are measured and quoted for Random Incidence Sound Reflections.
The reflections in a studio, especially from the side walls, are mostly at grazing angles. Under these circumstances many materials can appear reflective to the higher frequencies and hence their performance will be below that expected.
Listen to the Music not the Room is an article written by Dr. Peter D’Antonio of RPG Difuser Systems